Whittled down to the bone: developing a miniature in vitro bone model
With a decade of NC3Rs funding, scientists at the University of Birmingham have developed the first mature bone organotypic model, then systematically adapted and modified it to replace the use of rodents across a range of therapeutic areas involving the study of bone growth and regeneration. The models accurately recapitulate human bone development and physiology and are being used in labs in Birmingham as well as elsewhere in the UK and internationally.
First in vitro 3D organotypic model of mature bone
Bone loss and regeneration are typically studied in rodents through ovariectomy-induced osteoporosis, applying trauma to a tissue or fracturing a bone but these methods are associated with significant suffering. In addition to the animal welfare implications, rodents have different bone physiology to humans including different metabolism, lower volume of bone formation and differing mineral composition. Existing in vitro models were limited to simple 3D cultures or cultures of immortalised cell lines as mature bone cells, osteocytes, were difficult to differentiate and maintain in culture. These models failed to recapitulate important aspects of bone physiology, such as a relevant 3D structure or markers of mature bone growth.
Dr Alex Iordachescu developed the first in vitro 3D model of mature bone during her NC3Rs-funded PhD in Professor Liam Grover’s lab at the University of Birmingham and was able to culture these constructs for extended periods (over 1 year). Rat osteoprogenitor cells are embedded into a tissue scaffold which is held between two calcium phosphate anchors and when supplemented with cells, the system self-organises into osteonal structures that closely resemble mature bone in terms of chemistry (e.g. the collagen/mineral ratio) and cellular composition (e.g. osteoblasts and osteocytes are present). Growing the cells between fixed anchors provides alignment at multiple scales, mimicking the organisation of bone tissue and how matrix is formed by cells, something that is directly related to bone structure and function in vivo1,2. These constructs were also shown to be a source of osteogenic particles involved in mineralisation3.
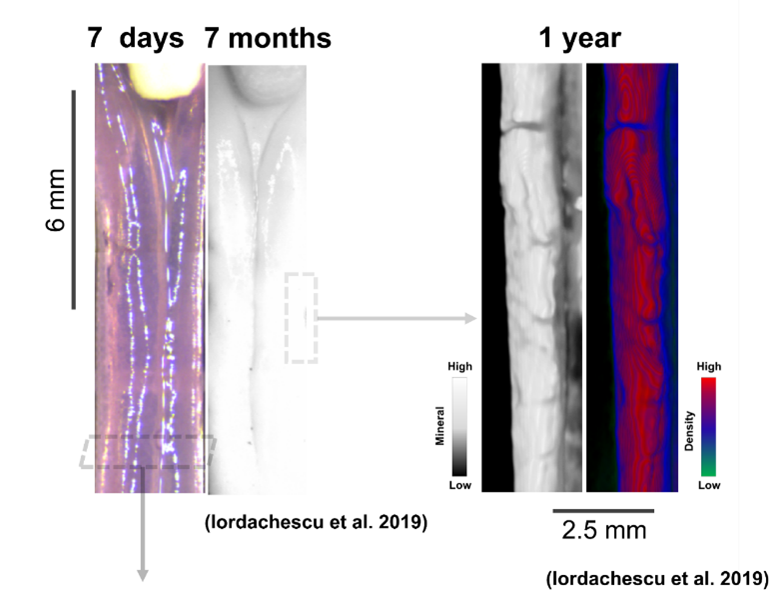
A humanised model of bone remodelling and geometrical adaptations
With a strong commitment to the 3Rs and keen to apply the bone model to study bone remodelling, Alex was awarded an NC3Rs Training Fellowship to adapt the system into a miniaturised, humanised bone organoid. The model was designed to be compatible with NASA-Synthecon bioreactors, which could provide a state of simulated microgravity/reduced mechanical stress enabling Alex to study the effects of unloading on bone remodelling. These developments also increase the utility of the in vitro method by enabling more high-throughput studies to further replace animals in bone research.
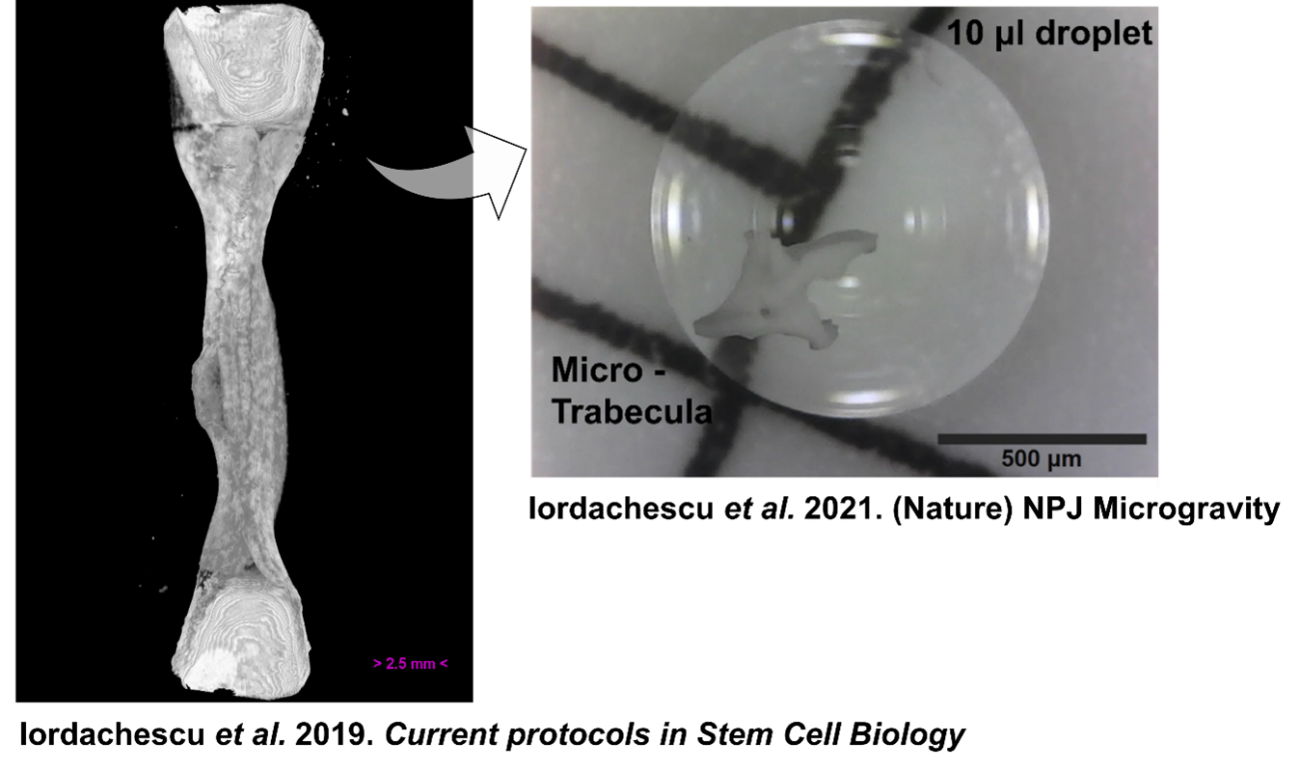
This humanised model used both osteoblasts, which mature into osteocytes, and osteoclasts, which resorb bone. These three cell types regulate repair and remodelling in healthy bone. Cell mixtures were seeded directly onto small trabecular bone fragments and subsequently encapsulated into small human synthetic blood clots, a material typically used in surgery, to increase their clinical applicability. These trabecular organoids developed large osteocytic projections, matrix deposition and resorption of bone, which can be used to examine cellular processes and identify bone loss or growth patterns. Importantly, Alex developed the model using gender-matched cells, as female patients are more likely to develop osteoporosis, increasing the clinical relevance of the organoid model.
The organoids also fit into a 96-well plate, allowing for high-throughput assays used in pharmaceutical development/toxicological testing and are compatible with a range of other culture systems. When cultured in simulated microgravity, Alex observed several differences from normal constructs, including in resorption lacunae morphology induced by osteoclasts4.
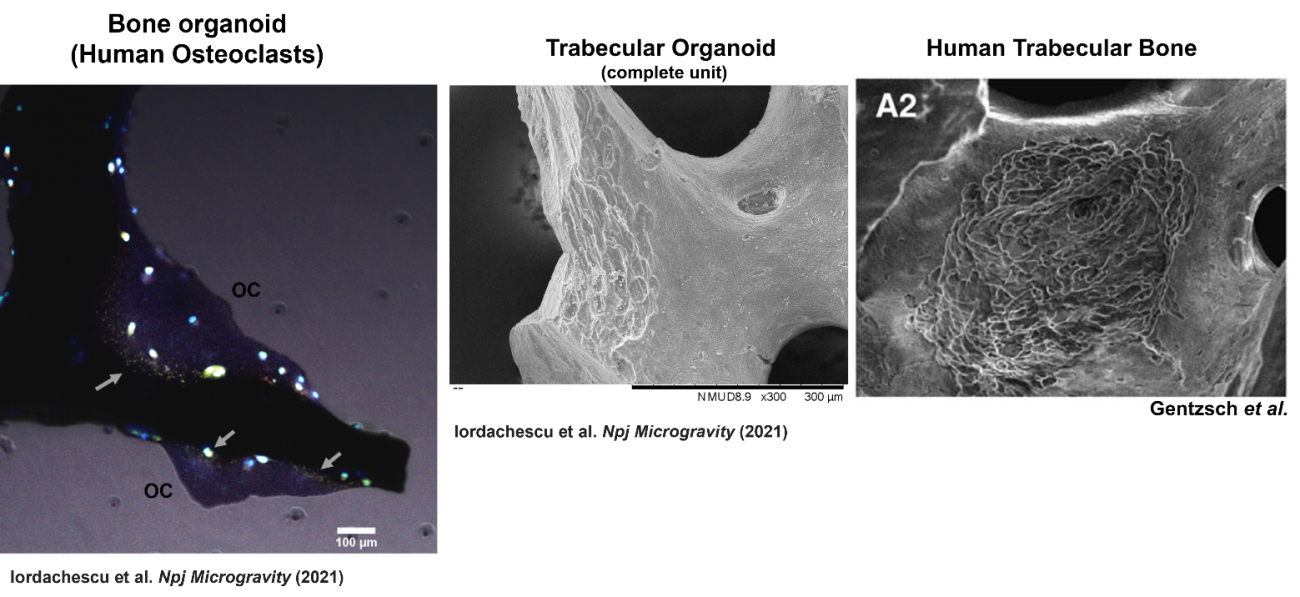
At the same time, Liam collaborated with another bone researcher at the University of Birmingham, Dr Amy Naylor to further develop the original organotypic model into an in vitro assay of bone remodelling. Amy was awarded an NC3Rs-funded PhD Studentship where Dr Melissa Finlay, the PhD student worked with Amy to adapt the method to use human cells and to also introduce osteoblasts and osteoclasts. The creation of a tri-cell organotypic model will enable study of bone remodelling in disease situations, such as osteoporosis, arthritis, and failure of fracture healing. This complements the organoid model in tools for studying bone remodelling as the organotypic model allows for disease to be studied with both self-organisation and 3D physiological human bone-like structure in an in vitro culture. So far, Melissa and Amy’s organotypic model of bone remodelling has directly replaced the use of 110 rats in their lab each year and reduced their reliance on mouse models of disease for mechanistic and early target identification work.
Applying bone organoids across bone research
The bone culture models developed at the University of Birmingham are replacing animal studies in labs both nationally and internationally. Liam, Alex, Amy and Melissa have established a series of collaborations, applying the bone models to research spanning from bone fractures to cancer and scoliosis.
Funded by an NC3Rs Skills and Knowledge Transfer grant, Alex began a collaboration with Dr Donald Ward at the University of Manchester to apply the trabecular organoid model to studies of bone endocrinology and mineral metabolism. Alex worked with Donald to use the organoids in a drug screening study, replacing approximately 25 to 30 rodents that would have been required and establishing the new method in the Ward lab.
Alex is also engineering the organoids and organotypic models for use in multiple bespoke culture and testing systems, to simulate significant mechanical stress such as in accidents, injury and significant trauma, which can result in fractures and affect surrounding tissues. The models are being applied to facilitate studies into a large number of skeletal conditions and response to extreme environments through the Consortium for Organotypic Research on Ageing and Microgravity that Alex established in 2022 during her NC3Rs-funded awards. This latest application of the bone cultures has the potential to replace significant numbers of the rodents, rabbits, dogs and non-human primates used in bone trauma research.
Simultaneously, the organotypic model was continued at Oxford University by Alex’s PhD advisor, Dr Philippa Hulley to allow co-culture of patient-derived human osteocytes with human osteoclasts and testing of bone anabolic drugs5. The work helped demonstrate how the mature primary human osteocytes in organotypic constructs secrete molecular markers during development and further validated the culture provides a stable and long-lived population of mature human primary osteocytes for a variety of research applications.
Melissa and Amy have also successfully driven uptake of the human bone organotypic model both within their institution and further afield. Professor Simon Jones at the University of Birmingham is using the model with primary human patient samples to study scoliosis. Studies to further understanding of how cells from different sites of the spine are affected by scoliosis were performed from osteoblasts isolated from teenagers undergoing spine corrective surgery. This work would not have been possible without the model. Melissa and Amy have published a methodology on the NC3Rs Gateway and shared their protocols with labs in Ireland and Germany to enable other researchers to benefit from them6. In a collaboration with the University of Muenster, Melissa travelled to Germany to teach the technique to Dr Corinna Wehmeyer and her group. Corinna is developing the model to include cancer cell lines and aims to reduce her lab’s reliance on animal models to study bone metastasis.
Amy and Liam are continuing to collaborate by working with industrial partner AstraZeneca to further develop the high-throughput screening capabilities of the model system. Amy has also been awarded an NC3Rs studentship that builds on the existing organotypic model – this project, led by Dr Gowsihan Poologasundarampillai (University of Birmingham) aims to produce a model of osteomyelitis (bone infection) to replace the need for animal models of this painful condition.
References
- Iordachescu A et al. (2018). An in vitro model for the development of mature bone containing an osteocyte network. Advanced Biosystems 2(2):1700156. doi: 10.1002/adbi.201700156
- Iordachescu A et al. (2019). Organotypic culture of clinically-relevant bone using composite ceramic-fibrin scaffolds. Current Protocols in Stem Cell Biology 48:e79. doi: 10.1002/cpsc.79
- Iordachescu A et al. (2018). A novel method for the collection of nanoscopic vesicles from an organotypic culture model. Royal Society of Chemistry Advances 8(14): 7622-7632. doi: 10.1039/c7ra12511a
- Iordachescu A et al. (2021). Trabecular bone organoids: a micron-scale ‘humanised’ prototype designed to study the effects of microgravity and degeneration. npj Microgravity 7:17. doi: 10.1038/s41526-021-00146-8
- Knowles HJ et al. (2023). Mature primary human osteocytes in mini organotypic cultures secrete FGF23 and PTH1-34-regulated sclerostin. Front Endocrinol (Lausanne). 8(14):1167734. doi: 10.3389/fendo.2023.1167734
- Finlay M et al. (2023). A detailed methodology for the long-term in vitro culture and analysis of three-dimensional, self-structuring bone models generated from cell lines or primary osteoblastic cell populations. F1000Research 12:357 doi: 10.12688/f1000research.130779.1